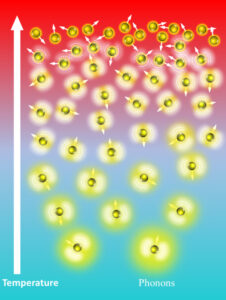
For decades, scientists have been trying to understand a discrepancy between theoretical predictions and what is seen in experimental observation regarding thermal conductivity at high temperatures. According to theory, thermal conductivity should drop much faster than it does in actual experiments. University of Utah mechanical engineering assistant professor Tianli Feng’s recent research has found an explanation for this discrepancy.
In solids, higher temperatures excite more heat carriers. The heat carriers (namely, phonons in the picture) in the solid become more crowded, and therefore, they are more likely to collide with each other, which generates more resistance to transporting heat. This is why solids show decreasing thermal conductivity with increasing temperature.
However, Feng’s research found that in solids heat carriers that would normally collide with each other are less likely to as the temperature increases. The collision cross-section becomes smaller at high temperatures. His findings were published recently in the latest issue of Materials Today Physics. You can read the paper here.
“If you think about the heat carriers as balls, although the number of balls grow with temperature, the ball size becomes smaller when temperature increases, making the balls less likely to collide with each other,” said Feng, pictured, left. “This is contradictory to what scientists believed before. As a result, the thermal conductivity of materials shows a much slower decay with temperature than previously thought. It can even increase with temperature! There are many other intriguing phenomena and unclear physical processes for us to reveal, and we are excited to disclose that our lab just received a National Science Foundation funding to specifically tackle these challenges at ultra-high temperatures in the coming years.”
This finding is significant as it provides a completely new insight into the behavior of thermal conductivity at high temperatures, and it will be broadly applicable across all crystalline materials. Because the development of many technologies requires an ultra-high temperature working environment, having better theoretical prediction capability will make a big difference. For example, next-generation gas turbines are desired to work at 1,300 degrees Celsius, much higher than the current 700 degrees Celsius, to boost efficiency and save energy. Higher-temperature working environment is also foreseen in next-generation nuclear fission plants, fusion power plants, and high-speed hypersonic passenger aircraft and re-entry vehicles.
All these technologies require effective management of heat flow at ultra-high temperatures. Feng’s findings will help inspire more new research in novel materials discovery and novel structure designs for ultra-high temperatures, and it can potentially lead to technology revolutions.